 |
 |
Phy.203 Links:
» Syllabus
» 203§2 web page
- - - Unit 1 - - -
» topic 1 (ch.20)
» topic 2 (21+23)
. . . here! ⇒
» topic 3 (22+23)
- - - Unit 2 - - -
» topic 4 (24)
» topic 5 (˝25+26)
- - - Unit 3 - - -
» topic 6 (25+˝18)
» topic 7 (18+19)
» topic 8 (17)
- - - Unit 4 - - -
» topic 9 (29+28)
» topic10 (30)
- - MU Physics 2 - -
» MU-online
(BlackBoard site)
» 204 §2 web page
» 204 §2 syllabus
- - Off-Campus - -
» PhysicsForums
(human hw help)
» hyperphysics
(detailed e-book)
» Knight 4th ed.
(pdf download)
|
 |
 |
 |
College Physics 2 (203) - Topic Two Assignments & notes
Science 159 (below 3rd Ave ramp) foltzc@marshall.edu don't phone - stop in!
Topic 2 (Electric Potential, Potential Energy, & Capacitance)
Readings for Topic 2 (Knight,Jones,Field 4th) : Ch.21 + 23§1 - 23§6
| 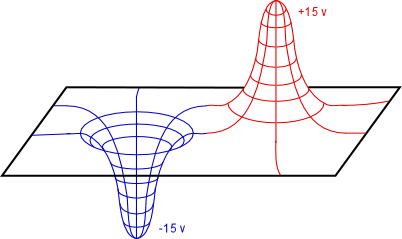 |
Quiz 2 − now to be Tue.Jan.30 − its header will look like this:
Physics II Topic 2 Summary
Static Electric Force is conservative, so Static Electric Field is a Conservative Field
"Conservative Forces" have a functional form that depends at most on (relative) coordinates - so the Work it does will always conserve Energy.
(unless the source itself moves in that coordinate system ... that would not be a "static" Force.)
. . . Conservative Forces allow us to formulate a Potential Energy function for them ... remind yourself how useful that was!
local (flat) gravity Force mg depends on no coordinate => Ulg = mgh , (height above surface) ;
elastic (spring) Force −ks is a f'n of stretch s along the Force, from relaxed size => Usp = ˝ks² , (radial stretch)
planetary gravity Force −|m GM/r²|^r is a f'n of radius from source center => Ug = −m GM/r , (radius)
fluid Pressure Force P A is not a f'n of any coordinate => Up = P V , (A·L = Volume).
. . . If a Force's function depends on the test object's velocity, then it can have non-zero circulation - and is not conservative !
viscosity Force −ηvA/d , and drag Force −˝ρv v·Acd , depend explicitly on velocity ;
kinetic (dry) friction "μkN" looks okay, but as a vector is actually −|μkN|^v ... opposite the velosity.
If a test object is moved in a circle, their Force arrows there (at places on the path) "circulate" opposite that path,
and they have done non-zero total Work, even as the object returns to where it started.
=> These Forces do not have a Potential Energy function ... their closed-loop Work is not zero.
. . . Some Forces depend explicitly on time (say, those exerted by animate subjects ... people choose to push, then quit pushing)
obviously the Work done by these cannot be written as a Potential Energy function in location (maybe a Power function in time)
If we abbreviate all these as "NPEF" , for No Potential Energy Formula :
=> Σ Einitial + Σ FNPEF ·Δx = Σ Efinal . . . is the Energy-conservation equation .
The Static Electric Field carried by charges (along the Force it applies to a "test" +q) always starts on (+) charge and ends on (−) charge
. . . the geometry wording says that E-field lines diverge from + charge, and converge to ( = − diverging from) − charge ;
so they have zero "circulation" ... even for dipole arrangements where the E-field lines curve.
=> There is a scalar function Ves (& also a Ues), whose gradient is the (negative) Electric Field (or Electric Force).
Suppose a big charge +Q is fastened in place, so the E-field it carries does not change.
1) As we move a (+q) test object slowly into the E-field, that Electric Field's Force does negative Work to the charge
while we do positive Work to the charge ... we push it from big distance r1 to small distance r2.
The object's ΔK =0, so the Work we did went into the Electric Field : Wby us = Faverage · Δr
2) Moving the object around Q at distance r2 , that motion is across (_|_) the E-field
. . . the field does zero Work (a dot product multiplies the vectors' parallel portions), and we do zero Work;
notice that our charge needs to stay at the same small distance so that its motion is always _|_ to the E-field.
3) As we move the object along the field, the Electric Force does positive Work to the charge, while we do (−) Work.
moving it from small distance r2 to big distance r1, the Work done is exactly opposite step 1's Work.
4) to arrive at the test charge's starting point, we move it sideways, (_|_) across the E-field ; having done zero Work.
| |
That is what it means to say that a Force is conservative
. . . the Work done by us against the E-field during step 1, is returned to us from the Electric field during step 3.
=> the Energy we transferred to the E-field was stored by it as electric Potential Energy, to be "given back" later.
Just like in Physics 1, we will define a Potential Energy function Ues so that
=> ΔU/Δx = − Fes,x ... similarly for the y-component and the z-component .
. . . this guarantees that the Work "not yet done" by the electrostatic Force , is still stored in the electrostatic Potential Energy
Fes· Δr = −ΔUes . . .
. . . notice: both sides of that equation contain the object charge q . . . when they cancel,
the resulting equation is only about the environment ... (like happened so often with object mass in Physics 1)
=> Ees,x = −δV/δx ... the Electric Field points toward lower electric Potential
. . . with no object referred to, nothing is really changing ... so Δ "time-wise change" is replaced by δ "place-wise difference":
Es· δr = −δVs . . .
Idea #4 - - - Charge Q is Source for Electric Scalar Field (Potential), V - - -
#4.a: Source (subject) Charge carries an Electric Potential - - - abbreviated V , units [Volt] - - - - - - - -
Nonzero subject charge Qs modifies the environment near itself ; Electric Potential surrounds it
V is positive near a positive charge ... V is negative near a negative charge
#4.b: Extreme Potential V nearby ... negligible V far away ... goes like 1/r - - - - - - - - - - - - - -
=> V = kC·Qsource/r .
r is the distance from the source charge Q to the place where the V is calculated
kC = 9E9 Vm/C . . . notice that 1 Volt = 1 Nm/C = 1 Joule/Coulomb
Every source charge (nearby) contributes to the Potential field at some location ;
. . . simply add all the contributions, algebraically (keep track of + or − signs)
of course each charge is divided by its own distance from the place of interest .
since V is a scalar, there are no vector components to worry about !
. . . charges that are not "nearby" don't contribute to V ... kQ/∞ → 0
Potential V (vertical scale, on 2-dimensional surface) caused by a charge dipole ...
. . . Potential at the right is positive (looks like a "hill"), so the (+) charge must be on the right
the negative "well" (on left) is centered at the (−) charge.
the Potential V = 0 , midway between the charges
. . . because the (+)Q's contribution is canceled by the (−)Q's contribution
in contrast, recall that the E-field is very intense there
. . . just like the mechanical Force was the (−)slope of the PEx graph ,
=> the E-field is the (−)slope of the potential graph
| 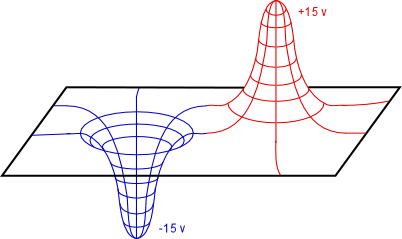 |
Idea #5: - - - Object charge q in an Electric Potential has Potential Energy - - -
#5.a: the Electric Potential V Energizes charge q - - - - - - - - - - - - - -
When an object with charge q is immersed in an environment with Electric Potential,
it has electric Potential Energy ... 1 Joule = 1 Coulomb·Volt
=> PEof q = q V . . . similar to gravity's PEof m = m (gh) = m (−GMEarth / r )
. . . this influenced charge {lower case} does NOT contribute to the V at its own location
(it's a passive object, not an active subject ... an object cannot give itself Energy ... we cannot divide by zero distance)
Positive Potential Energy means that our object charge is being repelled by the subject charges (which made the V there)
since they must have the same sign (−q in a negative V region, or +q near a positive Q)
. . . positive charges are always "trying to move toward" lower (−) potential ... (to be closer to negative charges)
while negative charges are always "trying to move toward" higher (+) potential
Suppose we stick a proton somewhere near that charge dipole (graphed above)
. . . because the proton has charge +e , its Potential Energy (qV) graph would identical to the V graph
simply change the vertical axis units from [V] to [eV] ... pronounced "ee vee" ... [1 eV = 1.6E−19 CV ]
. . . it would take Work from us , to roll it very far up that tall and steep "hill" (positive PE)
(the Work we did to the proton would increase its PE)
. . . but it would go faster and faster (Kinetic Energy) as it fell into the PE "well" (negative PE)
(if it didn't hit anything at the bottom, the proton could come up the other side, and out of the well)
Negative Potential Energy means that the object charge has been attracted to the Source charges
. . . unless its Kinetic Energy is large enough (so that its total Energy is positive), they're trapped by each other
since they cannot become "very far apart"
... at ∞ distance their U must be 0 , and KE = ˝mv² cannot be negative.
. . . an (−)electron gets trapped by a (+)proton if the electron loses some KE as it "falls up" the (+)V hill made by the proton ;
meanwhile, the proton looks like it falls down the (−)V well caused by the electron .
. . . the total PE is either: qelectron Vproton , or qproton Velectron ... do not "double-count" the Energy !
=> PE = ˝ ( qelectron Vproton + qproton Velectron ) . . . generally, ˝ Σ (qi Vj≠i ) , adding all charge pairs
- - - Vector and Scalar Fields are Related - - - −F·δx = δU - - -
This big idea from Physics I implies that the Electric vector field is also related to the electric scalar field , via Work
δV = Vb − Va = Vba is Related to the Electric Vector Field - - - - - - - - - - - - - -
The difference in Potential between one location and another , δV , is given by −E·δx .
. . . δx points from starting place to ending place (almost like a displacement)
=> If Electric Potential difference is thought of as "g·h" on a topographic map, then
. . . E would be the slope pointing in the steepest "downhill" direction , as the gradient of V ... δV/δx
OR => we might think of the Potential difference (=voltage) as being the "E-field accumulated along the path"
which is thinking of the E-field as a voltage spread out over a distance"
. . . this follows from the Work that would be done by the E-field on a charge which traversed that path.
Within a conducting material, the (static) Electric Field is zero , so E·dr = 0 .
=> Electric Potential V is a uniform value all through that conductor.
NOTE : "Voltage" means Potential Difference ... difference in Potential (δV) at two places (say, a and b) at the same instant
when written with 2 subscripts, Vba means the Potential at the first (b) , relative to (minus) the Potential at the second (a) .
. . . voltage is NOT the Potential at one location , at two different times (that would be a change in Potential "ΔV" )
. . . to be meaningful, "voltage at a location" must be relative to the "0" Potential at infinity, or specified as somewhere else.
=> "voltage across" means Potential at one side of a device, relative to the Potential at the other side of the device .
"voltage drop" envisions a path from high-potential side to low-potential side ... like waterfalls down a cliff.
Niagara falls' gravity potential drop is g δy = (9.8 J/kgm)(187m − 132m) = 540 J/kg ... down is a "positive drop"
Implication ... C = Q/δV - - - - - - - - - - - - -
conducting geometry has Capacitance to store Charge
Capacitance C = Q/δV ~ κA/4πkcd
. . . this is well-defined since any conductor has only one value for its potential (at any time)
. . . large surface Area A has large Capacitance property ; a lot of charge can spread out on it
. . . d is the distance along the E-field , a "length scale" of the conductor
small d implies that δV is small"close to zero" , which makes the capacitance large
Capacitance depends on Geometry and material properties - - - - - - - - - - - - - -
the charges carry the E-field which determines the voltage, so the ratio of them is only size and geometry .
. . . but di-electric material in the gap becomes polarized, which reduces the E-field within it, to 1/κ of the external Field
=> voltage is reduced for the same amount of charge , so capacitance is increased (κ × Co)
. . . many dielectric materials can withstand more intense E-fields before breakdown ("spark thru material") than air can,
so the gap can be thinner (which decreases the voltage even more, for the same charge on the same Area).
Capacitors Store Energy : Σ Q(t) δV(t) while being charged - - -
Because the voltage across a capacitor changes as the charge accumulates on it (or leaves it),
the PE stored in a capacitor is PE = qtotal·δVaverage = ˝ qtotal·δVmaximum .
... same sort of averaging process as storing PE in a spring : (˝ Fmaxxmax = ˝ k x² )
This implies ... since δV = Q / C ... and C is constant while being charged ... that
=> PEstored = Qtotal Vaverage = ˝ Qmax Vmax = ˝ Qmax²/C = ˝ C δVmax² .
Capacitors in Parallel : Q's add ... Areas add - - -
Charge is conserved, so the total charge from the battery splits up at a junction ;
more of the charge goes into the branch with the larger Area (if the gap distance is the same) ... proportional to that C .
This implies ... since Q = C δV ... and δV's are the same ... (A = C d 4πk / κ)
=> Cparallel = C1 + C2 + C3 + . . .
here, 1 F + 2 F + 5 F = 8 F
. . . and the 2F capacitor receives 2/8 of the total (battery) charge.
| |
Capacitors in Series : δV's add ... gap lengths along E add - - -
Energy is conserved, so the total battery voltage is divided among the gap distances ;
more voltage is across the Capacitor with the longer gap distance (if Areas are the same)
. . . each Voltage is proportional to 1/C , but they add up to the battery voltage.
This implies ... since δV = Q / C ... and Q's are the same ... (d = 4πk κ A/C)
=> 1/Cseries = 1/C1 + 1/C2 + 1/C3 + . . .
here, 1/Cseries = 1/1F + 1/2F + 1/7F = 1.7 1/F ... so Cseries = 0.588 F
. . . and the 2 F capacitor's voltage is 0.588/2 = 29% of (×) the battery voltage = 1 Volt
| |
Ignore This Section Until Comfortable with the Rest of Topic 2
Idea # 6 : the Electric Field itself implants Energy to the Volume of Space it Occupies
The PE of a collection of charges and fields is just that.
. . . It is shared among all of the entities in the system,
not just kept by the "test charge" in the V produced by the other.
. . . this is more important in Electricity than it was in Gravitation, since
there, a jumping person moved a long way, so measurable Work was done to them,
. . . while Earth moved a tiny distance, with opposite Force applied, so negligible Work was done to it.
here, it is often somewhat arbitrary which charge is thought of as "source", and which is called "test".
. . . In perhaps the best conceptual sense, Electric Energy is held in the Electric Vector Field
as an Energy density everywhere that the E-field is non-zero.
the "Field Energy density" is a Potential Energy per Volume , it can be called ρEnergy , or u .
to get a scalar, the vector must be squared ... to get units right, that must be divided by Coulomb's constant k:
=> Energy/Volume = ˝ κ ε0 E·E , if the region has dielectric constant κ .
. . . try writing the Gravitational Field Energy density, by analogy ... check the units!
Example: an electric rat cage, with 150,000V/m filling its .5m×.4m×.3m Volume, has
ρEnergy = (150E3N/C)(150E3V/m) / (8π 9E9Nm²/C²) = 0.0995 J/m³
. . . so it contains Energy U = ρEnergy V = (0.0995J/m³)(0.06m³) = 0.00597 Joule in field Energy
... (the power supply needed to provide 2× that much Energy, if its voltage was not adjustable) - but you already knew that, right?
A wondrous factoid: the E-field caused by a dipole spreads out
so that the intense field between the charges can weaken, and so reduce the total field Energy;
if E spread any farther, then the bigger Volume occupied would more than offset the reduced center intensity
=> the functional form (shape) taken by any static field will minimize that field's total Field Energy
(the very modern reason that opposite charges attract, is to reduce their total field Energy, by Volume reduction)
Example: Charged Conducting Shell U ... 3 ways:
method 1, considering the U as Work done to assemble the charges onto the shell, from their starting place at ∞
U = ˝ q V , where V = − ∫ E·dr ... from infinity in to the shell radius R .
E(caused by Q) = (1/κ) kc Q / r² (away) , so V = − kc Q / κ ∫ (−)dr / r ²
=> U = ˝ q (kc Q / κ R) ... = ˝ (kc/κ) Q ² /R .
method 2, considering the charged shell as a Capacitor :
U = ˝ Q ² / C ... C = κ ε A / d
A = 4π R ² ; d = R ... so C = κ ε 4π R => 1/C = kc/κR
=> U = ˝ Q ² kc/κR ... (same formula as method 1 )
method 3, considering the U to be contained in the Electric Vector Field's Energy density :
U = ∫ u dV = ∫ u(r) 4π r ² dr , with E = (kc/κ) Q / r² , for r > R .
. . . = ∫ (κ/8πkc) (4π r² dr ) (kc Q /κ r ² )² ... = ˝ kc Q /κ ∫ dr / r² ... we integrate from R to ∞
. . . (this Energy density is positive everywhere, so total U must be positive even if we integrate from ∞ inward to R )
=> U = ˝ Q ² kc/κR ... again ...
This seriously limits how small protons and electrons can be
An electron carries its E-field with it, where-ever it goes ... its E-field Energy acts as if it has inertia ... mass = U/c² .
. . . if all an electron's inertial mass was due to its E-field Energy , mc² = U = ˝ kc e² /R yields a minimum Radius
for the electron's charge , called the "classical electron radius" , presuming it is a shell
=> Re,E ≥ ˝ kc e² / mc² = 1.4 femto-meter ... 12% bigger than a proton or neutron (40% more Volume).
. . . if its charge is spread within its Volume, there would be E-field Energy inside its radius also
- so R would need to be 3/2 × larger (2.1[fm] radius)
. . . if some inertia comes from g-field Energy (Physics I), or from B-field Energy (Unit 2) , the radius must be larger again
=> a "point charge" would have infinite E-field Energy density, so cannot really occur .
. . . if some fraction f of its inertia came from "material mass" (whatever that would mean), the radius would need to be even larger still .
yes, the gravitational field contains Energy density in an analogous form
=> Energy/Volume = g·g/8πG . . . (with no negative masses, gravity has no "polarizability" κ )
. . . this is not included in Newtonian gravitation (Physics I), but is the key new feature in General Relativity (Unit 4, maybe)
. . . the Field-Energy density acts like an "effective mass density" ... ρm_field = (U/Vol)/c²
at Earth surface , ρm_g-field = (9.8N/kg)(9.8m/s²)/(8π6.7E−11Nm²/kg²)/(3E8m/s)² . . . = 0.63 milligram /m³
(it becomes noticeable in the intense g near compact stars, and for the immense Volume of the entire Universe).
For comparison ; a typical 100 N/C Earth E-field has Field-Energy density E·E/8πk = 44 nanoJ/m³ , so ρ/c² = 49E−24 kg/m³
|