 |
 |
Phy.203 Links:
» Syllabus
» 203§2 web page
- - - Unit 1 - - -
» topic 1 (ch.20)
» topic 2 (21+23)
» topic 3 (22+23)
- - - Unit 2 - - -
» topic 4 (24)
. . . here! ⇒
» topic 5 (½25+26)
- - - Unit 3 - - -
» topic 6 (25+½18)
» topic 7 (18+19)
» topic 8 (17)
- - - Unit 4 - - -
» topic 9 (29+28)
» topic10 (30)
-- MU Physics 2 --
» MU-online
(BlackBoard site)
» 204 §2 web page
» 204 §2 syllabus
- - Off-Campus - -
» PhysicsForums
(human hw help)
» hyperphysics
(detailed e-book)
» Knight 4th ed.
(pdf download)
|
 |
 |
 |
College Physics 2 (203) - Topic Four Notes & Assignments
Science 159 (below 3rd Ave ramp) foltzc@marshall.edu don't phone - stop in!
Topic 4 (Magnetic Field & Sources; Force & Torque)
Readings for Topic 4 (from Knight Jones Field) : Ch.24 + Ch.25§2
| 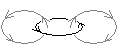 |
plan for Topic 4 Quiz to be Tue.Feb.20 - its header will look like this:
Physics II Topic 4 Summary
observations about permanent magnets, and their implications:
every permanent magnet is a dipole ... North pole and South pole, on opposite ends.
* nearby opposite poles attract, nearby like poles repel ... especially long bar magnets
=> so "naturally oriented" dipole magnets attract ... especially short button magnets
* magnets also turn (rotate) thin iron needles ("filings"), and attract them, even if they are not permanent magnets
. . . the spatial pattern strongly suggests that a magnet must be surrounded by a magnetic vector field
=> the magnetic field will be parallel to the filings (but a vector must point − which way?)
* the compass needle's "Northward-pointing end" defines what was called the "magnetic North" pole
. . . and the compass arrow's pointing (arrow) defines the orientation direction for the magnetic vector field.
=> the magnet's magnetic field, outside the magnet, points toward a "magnetic South" pole, away from a "magnetic N" pole.
. . . Earth's "magnetic South pole" is now beneath the Arctic Ocean (almost Siberia), near Earth's "geographic (spin) North Pole.
* if a permanent magnet is broken, 2 new poles (N & S) appear at the (new) broken ends
=> magnetic monopoles (analogous to mass or electric charge) do not exist
. . . so we cannot compute a magnetic dipole vector like we did for an electric dipole (d = ΣQx)
. . . with no "magnetic charge" to be conserved, there is nothing analogous to a "magnetic current".
there is no magnetic topic that is analogous to "electric Topic 3"
* the magnetic torque applied to a compass needle increases with offset angle (from its stable equilibrium orientation)
. . . at small angles, the compass needle oscillates around that equilibrium at near-constant frequency
=> the magnetic field vector's intensity can be measured by that f ... [ ω = 2πf = √(τ/θ/I) ]
. . . similar to how a pendulum's frequency can measure a gravity field intensity
. . . but we would need to know (be able to figure out) the compass needle's magnetic dipole moment !
* if a hole is drilled in a permanent magnet, and a small compass is inserted into the hole,
. . . the compass points from "S" end to "N" end (oscillates with very high frequency)
. . . (is more intense in the middle of the magnet than at the ends)
=> the magnetic field "lines" have no divergence ("start" or "end"), but "loops" thru the magnet material
. . . so the dipole's magnetic field lines are shaped different than an electric dipole's electric field lines
Describing Earth's Magnetic Vector Field ... symbol B, measured in Tesla [T = N/Am] (=10,000 Gauss)
Vector arrow exits a magnet's "North" pole ... enters it's "South" pole
. . . Earth Magnetic "North" pole is under Antarctica ... a compass N pole is repelled by it, to point "geographically Northward"
the B-field points Northward (horizontal) above the equator
. . . Earth's "Magnetic South" pole, from N spin pole surface, is ≈ ½-way to Earth center (Florida-Pakistan line);
so Earth's B-field dives inward here ... inclined 60° below horizontal on Gulf Coast, "dips" 70° in Cleveland.
. . . intensity near Earth surface is about 52 micro-Tesla [μT] here ... 1 Tesla is really stiff magnetic field.
denser B-field near the poles (65 [μT]), sparser near equator (25 [μT]), where B lines spread into space
... (yes, B intensity goes as the magnetic "field-line" density, just like the g-field and E-field.)
. . . total "magnetic flux" B·A thru Earth's northern hemisphere surface Area is about (−)3E9 [Tm²]
total magnetic flux out from Earth's southern hemisphere surface is about 3E9 [Tm²] ... (out is +)
... total magnetic flux above Earth's equator (plane extended into space) is about 3E9 [Tm²] ... (crossing northward)
. . . Earth's Magnetic South pole is now near its geographic (spin) North Pole
. . . but our B reverses ("flips") routinely (avg half-million years), and is overdue (>¾ Myr).
. . . the flux centroid is moving fast lately (220 km last year), and seems to be fragmenting into 2 or 3 concentrations
The rest of Topic 4 summary will first treat B-field causes (production), building from single-particle sources to practical lab devices ;
. . . then deals with its effects (measurement), advancing from a single particle to less direct (more complicated) effects.
this is opposite Knight's presentation order ... and shows more right-hand rules (differently)
Idea # 8: "Charge in Motion" is encircled by Magnetic Field B - - - - - - -
As a (+) charge Q moves away, relative to the observer, the Magnetic Field B points in a clockwise sense
. . . the B-field is stronger for more charge, and for faster motion Qv
think of Qv (one entity) as the Source of its Magnetic Field
. . . the B-field is strongest close to the moving charge Qv
decreasing as 1/r ² at large distances r from an individual source charge
. . . the B-field is strongest in a "ring" around the moving charge, 90° from its motion "axis" along the Qv vector .
decreasing as sin(θ) at other orientations ... so is zero directly in front of Qv (and zero behind it).
=> B = (kmagnetic) Qv × ^r /r ² = (kmagnetic) Qv sin(θ)/r² . . . the "Biot-Savart" equation
. . . here, ^r is the unit vector in the direction toward the "field point" (place of interest)
. . . and the "magnetic Units constant" kmagnetic is usually written μo/4π (recall gravity's G, and electricity's kc)
. . . in SI units , μo = 4π × 10−7 Tesla.meter/Amp . . . exactly (from the old definition of "Ampere")
| 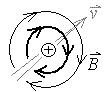 |
This cross product "×" uses the same right-hand-rule as was introduced in Physics 1 for torque : r × F = τ .
. . . with right arm or wrist aligned with the r vector (extends from rotation axis to Force application point) ,
fingers flip to align with F vector (sweeping thru angle φ ... that letter φ is spelled "phi" but pronounced "fie") ;
=> Thumb points | to r and | to F , in the direction of the τ vector , with magnitude r F sin φ
making B . . . carpals along Qv (think "current", remember topic 3) ... forward, in the line 1 example
. . . fingers flip thru φ, to point along ^r toward the field point (say, to the left)
... thumb points in the magnetic field direction ("missing middle falange"??) at the field point (left of forward) ... "up" (good)
It is often useful to think of magnetic Field lines, which encircle (as closed loops around) the Source charge as it moves
with arrows along the loop to show the B-field direction there
. . . in this case, right hand grabs the moving charge with thumb pointed along Qv , and fingers wrap along B lines .
this method ("encircle the piercing vector") follows the geometry introduced for spin and angular momentum :
. . . align right thumb in the direction of the angular momentum vector, along the spin axis L = r × p
right fingers wrap around the axis in the direction of the momentum for the individual pieces.
Add the Magnetic Field contributions from all nearby moving charges - - - - - -
If the moving charges are isolated (discrete, ~ far apart), be sure to get each one's B-contribution as a vector first,
before adding those contributions (as vectors) ... yeah, it gets quite tedious after about 2 sources (moving charges).
Unless there are a LOT (10²³) of them ... lined up nicely ... moving at the same (average) velocity ...
. . . A line of charges moving at the same velocity (along the line) appears to be a current along the line's Length .
=> the symmetry allows some important "special case geometries" to be calculated (even without integral calculus)
Geometry & Units: Q v = I L - - - - - - - - - - -
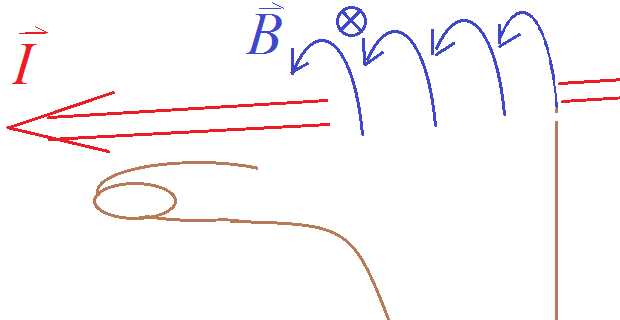
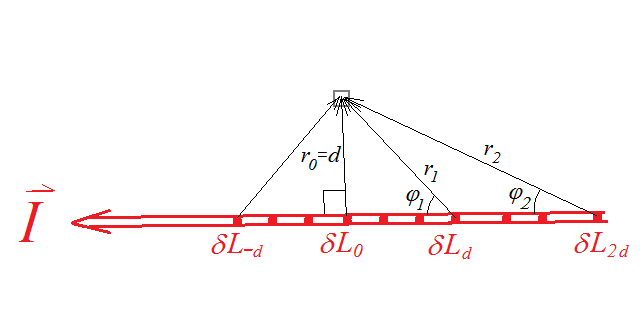 |
Current flowing along a Long, Narrow, Straight wire
A straight line of charge moving along its length is encircled by B (clockwise as seen from behind them)
same amount of "magnetic flux" pierces out of page below IL (for IL to the left) , as pierces into page above it (B lines form closed loops!)
. . . they appear as a current ... each moving charge contributes to the magnetic field everywhere else;
especially places close beside it ... so, B at some place (the field point) is mostly contributed from nearby sources.
When you sum (or integrate) the Biot-Savart formula for the B-field contributions from all these moving source charges
(treat each piece of charge as δQ ; presume positive ... if so, each contributes B into the page)
. . . if you know the current, you want to treat each as a piece of current (δL long) by replacing δQ v by I δL .
for a field point at distance d from a very long Line, the "effective Length" for an infinitely-long Current , is only 2d ,
that is, the only effective charges are from −d to + d ... at these limits, the radius r1 = d √2 (so 1/r² = ½ ) and sin φ = .707
so they only contribute .353 (=35%) as much as the closest charge.
. . . B-field contributions from charges out to ±d , accumulate 71% of the total that an infinite wire would have.
the distant ones, all the way to ∞ , contribute just enough to make up for the "nearby" charges doing less than the closest one.
plug this effective length into the Biot-Savart formula (above); the 2 cancels, and the d cancels one of the bottom r 's.
=> B∞ line = μo I /2π d
. . . if the wire really is only 2 d long , the magnetic field is .707 (=sin 45°) this intense; 4 d long produces 90% =sin(Arctan(2))
|
If the Current is Spread Out ( | to Qv) , use Ampere's Law
Ampere dissects the formula just derived. At distance d from the Current Line, there's a magnetic field line encircling the Current Line;
the formula's denominator (2π d) is a circumference, which is the length of the magnetic field line there
. . . the numerator (μ I) is the total source current that pierces (goes thru) the disk Area inside that circumference
... Itotal is the current density j = ρv , times the Area A ... recall ρv·A from Unit 1
Ampere's Law has the perspective that the magnetic field is weakened due to it being stretched along this circumference ℓ
=> Σ B · ℓ (closed loop) = μo Σ Ipiercing ... .
Example: B inside a big wire (radius R), if its current is spread over its large Area (as a current density j = I/πR²)
. . . how much of the current pierces a smaller disk radius r within the wire? ... j π r ² ... that means I r² /R ² ...
B is stretched around its circumference 2πr , so B = μoI (r²/R²) /2πr = μo I (r/R²) /2π .
. . . at the wire's center , r = 0 ... but B is not ∞ , it is zero there.
... the Area (on top) gets small "sooner than" the circumference (on the bottom)
grows in intensity at larger radii inside the wire, because the current (thru the Area) grows faster than the circumference
decreases in strength as 1/r , outside the wire, because the circumference grows without catching any more current.
| 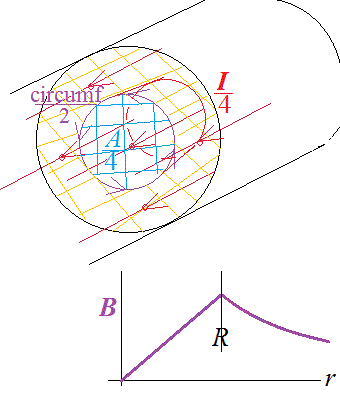 |
a Circular Current Loop concentrates its B-field inside the loop
add up Biot-Savart contributions (to making the magnetic field) from charges moving along a Loop
A Circular Loop of current is all the same distance from the center , and all μo Qsourcev are 90° from the r direction
(so sin(90°) =1) , and the effective length is the current loop's actual circumference.
=> BLoop center = μo I / (2 R) .
. . . a wire coil is usually treated as N loops , all in the same place ... use their average 1/R :
=> Bcoil = N Bloop
no matter how strong it is in the center, which depends on the radius R of the physical loop ,
the field must become more intense very close to the souce wire (see Ampere, above)
. . . but most of the Area inside a coil has a fairly uniform field .
same flux (B·A) up thru the inside of the loop (if current is counter-clockwise)
as there is down around the outside of the loop - spread over a large Area, B is weak outside.
. . . The magnetic field pattern due to a current loop is the same as that due to a permanent "button" disk magnet
=> North pole on counter-clockwise side, South pole on clockwise side
Not a coincidence ... permanent magnets ARE caused by (a LOT of) atom-sized current loops !
regular spacing of loops makes Magnetic Field nearly uniform within a Current Solenoid (Helix) - - - - - - - - - -
treat a solenoid as a series of loops with uniform spacing - - - - - - - - - -
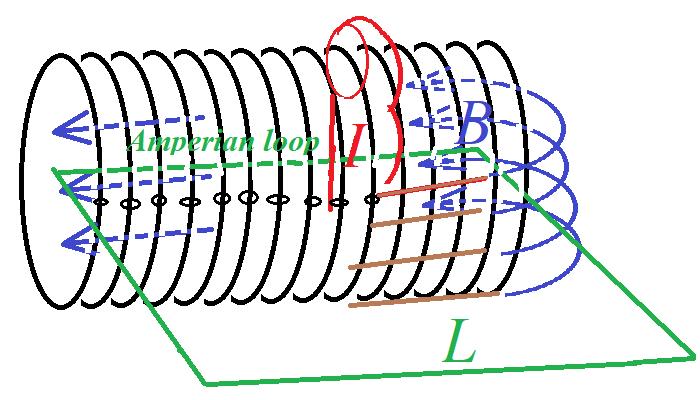 |
each loop carries current I (it's always the same wire in a helix)
. . . with N loops in length L, an "Amperian rectangle" from solenoid axis outward shows uniform B-field
until it extends past the wire loop radius and is pierced by the current.
. . . then, the length L inside the solenoid is pierced by N current loops ; Ampere says B·L = μo N I . . .
the rectangle length outside the solenoid can have essentially zero magnetic field along it, if we make it far from enough away from the solenoid
=> Bsolenoid inside = μo I N/L . . . outside, the field is nearly zero ... good approximation if not too close to either end coil.
. . . caution: N/L is sometimes abbreviated as n !
|
Idea # 9, What does Magnetic Field DO ? B deflects Moving charge - - - - - - - - - -
We measure magnetic fields by how hard they push moving charge ... units [Newton/(Cm/s)] is called a Tesla (recall Electic Field units [N/C] )
Magnetic Vector Field surrounds Magnets . . . symbol B, measured in Tesla [T] (=10,000 Gauss)
As a (+) charge q moves within a magnetic field, relative to the magnet, a Force is exerted on the moving charge
. . . the Force strength is proportional to the B-field , and proportional to qv
(...this symmetry between Source and Influence is necessary for Newton's 3rd Law to be true for Magnetic Forces ...)
. . . the Force is perpendicular to qv , and also perpendicular to B
(...recall that the B-field is perpendicular to its moving source Qv ...)
=> F = qv × B . . . Earthlings use a right-hand to obtain the direction for the force from this cross-product
(just like torque: r×F = τ ... rist × Fingers => thumb) ... qv ("carpals") × B ("manual phalanges") => F ("fumb")
just as with torque, the strength of the magnetic Force is q v B sin φ ... φ is the finger-flip angle, from velocity to magnetic field
| 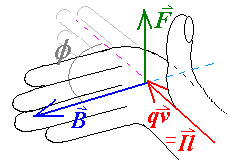 |
Magnetic Force tends to make freely moving charges travel in a circle
. . .recall (Physics 1) that Force perpendicular to velocity leads to a curved path , with radial acceleration component
a·r = −v·v . IF the magnetic Force is the only one acting,
=> r = mva/qBsinφ . . . velocity components along B and along v are unchanged.
Magnetic Field does zero Work to freely moving charges
. . . the Force is always | to the velocity (displacement is v Δt)
=> so there is no such thing as a magnetic scalar potential, and no "magnetic capacitor"
there is no "magnetic Topic 2" analogy
Add up the Magnetic Force applied to all charges moving within the object - - - - - - - - - -
Magnetic Force applied to a straight Wire, carrying Current
=> F = IL × B , where L is the Length of current immersed IN the magnetic field .
Total Magnetic Force on a Wire Loop is zero , if the external B is uniform.
Non-uniform Field can apply net Force to a current loop
. . . because the Force applied to opposite sides of the loop won't cancel
since current lengths IL are opposite but B is weaker at one of them.
. . . magnet attraction depends on the curl in the B-field (curl around z is δBx/δy − δBy/δx )
the x-component differs at various y-locations , and the x-component is oppositely different at varying y)
=> parallel current loops attract each other.
Uniform External Magnetic Field B Applies Non-Zero Torque to a Current Loop - - - - - - - - - - - - -
Recall (from Physics 1 or Topic 1) that Torque around an axis depends on the distance from the axis that the Force is applied ;
. . . τ = r × F since the magnetic Force is perp. to B , large Torque occurs where B || r ;
for a "rectangular" current loop, Force applied to length "L" ( | to B) causes Torque due to "lever-arm" width W ( || to B) .
=> τ = I A×B ... where the loop Area A = L × W (or any other shape)
A coil is a number N of loops, in series ... each feels the same torque (they carry the same current around the same Area).
. . . just add all the torques applied to each indicidual loop
=> τcoil = N I A×B ... the device property is its magnetic moment (N I A) ... the environment property is B .
notice that maximum torque orientation (A | B) has zero magnetic flux thru it (Φ is max if A || B)
Recall (from Physics 1) that Torque can do Work as the loop orientation changes : W = τ· Δθ
W = F·Δs , but in rotation , s is the arc length r Δθ along the circumference
. . . a current loop tries to align its interior B-field with the external magnetic field.
in that sense, the current loop has a PE which varies with orientation
. . . PE = − I A·B . . . it is minimum when Area (thumb, when fingers curl along I loop) is along B ;
it is zero when A is 90° from B ... and maximum when A is opposite Bexternal ... zero torque, but unstable (positive) PE.
. . . a coil made with N many current loops , is described by its "magnetic moment vector" μ = N I A
right-hand fingers curl along the current loop I that encircles the Area vector (thumb)
=> magnetic moment vector points along the magnetic field inside the device . . . from South Pole to North Pole, inside a bar magnet
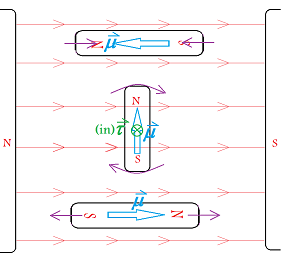 |
the B-field caused by a current loop is along that Area vector,
right-hand fingers circle along the current, thumb points along B vector
. .. so its "self-flux" = flux thru its own Area ... is positive μo μ·A/2R
. . . when the current loop is put in an external (environment) B ,
(top orientation) the environment's B-field might oppose the current's B-field inside the loop
where the Forces applied to the electromagnet "poles" compress the electromagnet
or (as on bottom) might reduce the magnetic field near the coil within its volume
where the electromagnet poles are "stetched" in Tension by the external B-field
. . . The torque (caused by the magnetic Forces applied to the electromagnet "poles")
does Work to the coil as the coil flips over (shown at center)
=> PE = − μ·B ... = − μ B cos θ . . . a scalar Energy, from the parallel components
. . . in this notation, τ = μ × B . . . a vector torque from the perpendicular components . . . NOT the same thing !
visualize the ±PE and τorque vectors in the external B-field in the diagram.
|
Atoms tend to align their own magnetic field with an external field.
the valence electron "cloud" for many atoms can be treated as a current loop ; if it can re-orient,
. . . the successfully aligned magnetic fields add up . . . especially noticeable in a "ferromagnet" material
=> magnetic field inside material is usually more intense than outside . . . in iron, is 500× -to- 2000× as intense
Electromagnet : coils make magnetic field, which aligns the iron atoms in the poles and the "yoke" (body between the poles)
the "lines" of magnetic field seem to be channeled within the iron;
the lines must exit the pole-tips "nearly perpendicular" ... sin θ ≈ θ [radian] ≈ 1/2000 ≈ 0.03° ... for good iron.
The Lorentz Force ... qv×B ... Separates + from − charges . - - - - - - - - - -
Mobile Charges Separate - via qv×B - - - - - - - -
. . . until the charge accumulated at the conductor's edges Q causes an Electric Field
whose Electric Force on any new charge q is strong enough to cancel the Magnetic Field Force.
. . . qE = −qv×B , so free charge remaining in the bulk material feels zero Force , and no more accumulates at the edges ... but
E ≈ − v×B all through the conductor , so large voltages might develop. If a conductor with width δx has qv along +y,
. . . δV = −E·δx = v×B ·δx called "motional EMF" , .
Notice that δx × v defines a rate of Area swept by the motion .
. . . this can be used to generate DC voltage and current , by spinning a disk which is pierced by a magnetic field
If (instead) current is pushed through such a device , it forces the disk to spin . . . a DC motor .
The E-field caused by the Charges (distributed at the object's edges) is accompanied by a Voltage
=> δV = −E·δs ... called the "Hall Voltage", when E is caused by current-carrying charges , separated by an external B .
Since only relative motion is meaningful, charge separation also occurs if a "moving" magnet is swept past a "stationary" conductor
Idea # 10 : the Magnetic Field imparts Energy to the Volume of Space it Occupies
The Magnetic Field B contributes to the total Field Energy Density in otherwise empty space.
=> Energy/Volume = B²/2μo . . . not B²/8π μo , since the magnetic source equation was written with μ/4π , not just kc .
It is this aspect that determines how the magnetic field lines spread out from some magnet :
. . . if they curl too tightly (from North pole to South pole), they would be very close together
so B² would make a very large Energy density (even though in a small Volume).
. . . if they extend too far from the magnet, they would occupy a very large Volume
even though the energy density would be very sparse .
=> Fields behave in a way that makes their Total Field Energy minimum . . . like any other Potential Energy .
of course Electric Fields and Gravity Fields behave the same way ... see Physics II's Topic 2 Summary (bottom of page).
|